Helge Bonesmo
Head of Department
(+47) 917 93 100
helge.bonesmo@nibio.no
Place
Trondheim
Visiting address
Klæbuveien 153, bygg C 1.etasje, 7031 Trondheim
Authors
Erlend Hustad Honningdalsnes Erik Stensrud Marstein Dag Lindholm Helge Bonesmo Heine Nygard RiiseAbstract
No abstract has been registered
Authors
Erlend Hustad Honningdalsnes Helge Bonesmo Erik Stensrud Marstein Steve Völler Heine Nygard RiiseAbstract
No abstract has been registered
Authors
Erlend Hustad Honningdalsnes Helge Bonesmo Erik Stensrud Marstein Steve Völler Heine Nygard RiiseAbstract
No abstract has been registered
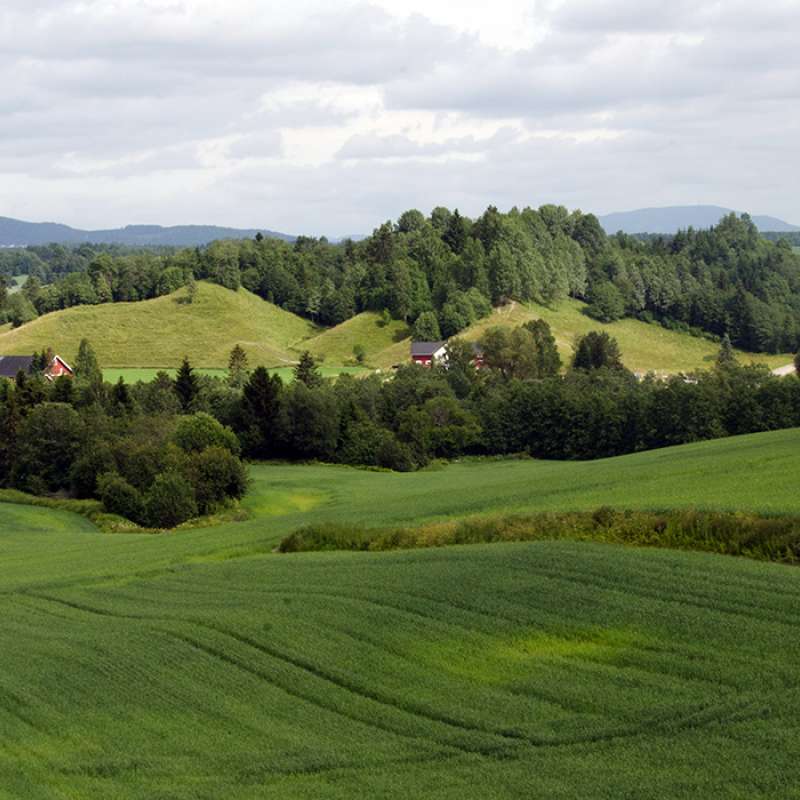
Division of Food Production and Society
Visions and the consequences - analysing visions for Norwegian agriculture and its consequences for food security
In the FOSIP project (Visions and the consequences - analysing visions for Norwegian agriculture and its consequences for food security) we will assess and evaluate the foundation, support, opportunities, and limitations for the goal of increased agri-food self-sufficiency in Norway and assess how far an increase will contribute to improved national food security.
.jpg?quality=60)
Division of Food Production and Society
Cultivating sustainable changes in livestock feed production and feeding practices (Feed&Feeding)
The project will evaluate various strategies for feed production and feeding practices to enhance the sustainability of Norway's food system and support national agricultural policy goals. These strategies include adjusting livestock diets, improving breeding and animal health, and introducing new protein sources for feed. The project will assess environmental impacts, such as land use changes, greenhouse gas emissions, soil carbon levels, nutrient balances, and biodiversity, as well as socioeconomic impacts, including food security, economic and social sustainability, and the viability of rural communities.